What Electric Fish Can Teach Scientists about NeuroAI
Modeling their behaviors may help in development of new AI systems
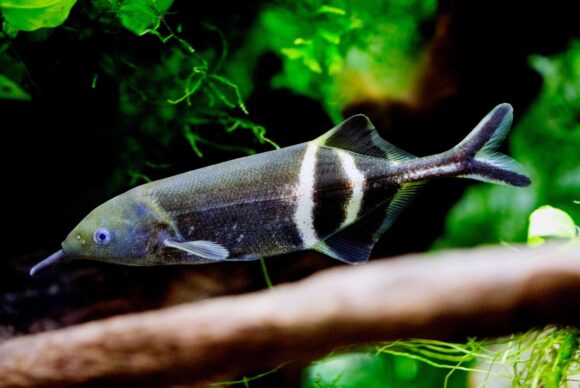
Elephantnose fish, named for the trunk-like protrusion on their heads, "see" their world by sensing electric fields and also by generating their own.
Electric fish are among the most intriguing specimens in nature’s cabinet of curiosities. They ‘see’ their world and each other by sensing electric fields – and also by generating their own. This unique ability provides a key area of exploration for the emerging field of NeuroAI, which explores the perceptual and cognitive capacities of both natural and artificial systems.
For Kanaka Rajan, Kempner Institute Investigator and Associate Professor in the Department of Neurobiology at Harvard Medical School, electric fish represent a potential source of wider insight into “collective intelligence,” a form of intelligence that emerges from interactions among different “agents,” or goal-oriented entities. Rajan and her team believe that studying weakly electric fish might prove to be a stepping stone on the road to understanding multi-agent intelligence, potentially shedding light on the complex dynamics that are a hallmark of animal and human societies.
There are several species of weakly electric fish. Rajan and her collaborators investigate the species Gnathonemus petersii, or Peter’s elephantnose fish, named for the trunk-like protrusion on their heads. These fish, native to the rivers of western and central Africa, prefer to live in muddy pools and shady, slow-moving streams, where vision is not always a helpful sense. Electro-sense helps them penetrate environments that are literally “clear as mud.” The fish don’t just sense electrical fields, they also produce them, with pulses known as electric organ discharges (EODs).
“They do everything in their lives through electric pulses,” says Rajan. “Their communication, their mating habits, their pursuit, their aggression, cooperation, competition, everything is governed by these pulses that they emit.”
Because Rajan and her team are interested in how agents – which could be humans, animals, robots, or language models – interact with each other at different scales, elephantnose fish offer an opportunity to study emergent, coordinated communication, or agent interaction, in a relatively simple context.
Agent interaction is, in general, an exceptionally complex research topic. Studying the roughly 86 billion neurons in a single human brain is challenging enough, and incorporating how brains interact with each other adds to the complexity. Human language and culture add yet another layer of complexity on top of that.
Here’s where the weakly electric fish come in: their “language” consists of streams of identical electrical pulses. Their “sentences” vary in terms of how often these “syllables” are emitted by each fish. But even though these animals have simpler forms of communication than humans, they still display emergent, coordinated behaviors that could shed light on much more complex social dynamics. Modeling these behaviors can also help in the development of new AI systems.
The key idea when studying collective intelligence is that social dynamics are not simply the sum of individual behaviors, or even of pairwise interactions. For example, the distinctive behaviors that can arise in groups of humans at, say, a party, are much more complex than a collection of two-person conversations. This could be because each conversation carries with it a memory remnant from the previous conversation, even if it occurred with an entirely different person. Or, because a three-way discussion has nuances that cannot be captured wholly by the sum of every possible two-person chat. Plus, the overall context matters when it comes to human behaviors – whether it is a work or an after-work event, for example, or whether the situation takes place between peers or within a social hierarchy.
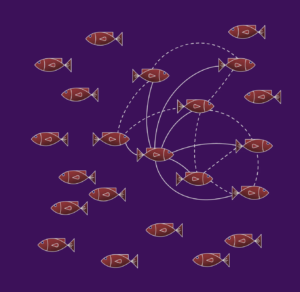
Elephantnose fish demonstrate this sort of collective intelligence in quite striking ways. As one example, in a study by Federico Pedraja and Nathaniel Sawtell, one of Rajan’s key collaborators at Columbia University, elephantnose fish in a group were found to be able to piggyback off each other’s ability to search for food. If one fish happens to find a promising source of food, it can send out pulses that nearby fish can detect. As a result, other fish can expend less energy on foraging directly and simply follow the leader. Through this and other types of collective communication, the fish are able to exhibit social behaviors that can help them survive and thrive in certain environments.
Rajan and her team build computer models using artificial agents that mimic elephantnose fish. By manipulating factors that would be impossible to control experimentally in real fish, Rajan and her team are able to simulate and study how collective intelligence emerges in different contexts.
Their work at the Kempner Institute has only just begun, but is already revealing some fascinating results. Evolutionary simulations – in which artificial fish must survive in order to pass on their characteristics to the next generation – suggest that the availability of food determines whether the fish engage primarily in cooperation or in competition. Reducing the reliability of food sources increases the likelihood that the population will engage in competitive behaviors. Cooperation and competition aren’t hardwired into these artificial agents. Rather, these behaviors emerge over the course of multiple generations through a simulated version of natural selection.
Rajan aims to expand this project to gradually encompass more and more complexity, including better understanding the process of learning in individual agents and the emergence of different survival strategies. “How do you run a whole society based on just pulses, a low-dimensional language, so to speak?” asks Rajan. “To me, that alone is intrinsically interesting.”
One key question the team is starting to address is whether there are universal laws governing social interactions. Is there, for example, a “critical mass” of agents that can optimally cooperate? Or a threshold after which competition becomes excessive?
This kind of work has intriguing implications for human cooperation, but is also relevant to applied AI research. Principles of collective intelligence may play an important role in how individual AI systems interact and communicate with one another. Cooperative teams of AI agents – sometimes referred to as “swarms” – are promising for tech applications, potentially allowing for faster, more complex, and more adaptable problem solving. Only time will tell if such AI “hive minds” prove useful, but in the meantime, foundational work of the kind pursued by Rajan and her collaborators is shedding light on how both biological and artificial agents can work together to produce collective intelligence.
About the Kempner Institute
The Kempner Institute seeks to understand the basis of intelligence in natural and artificial systems by recruiting and training the next generation of researchers to study intelligence from biological, cognitive, engineering, and computational perspectives. Its guiding principle is that natural and artificial forms of intelligence share important characteristics. Future innovations in artificial intelligence (AI) will take inspiration from the principles that our brains use for fast, flexible natural reasoning. In parallel, theories developed for AI will enhance our understanding of how our brains compute and reason. Join the Kempner mailing list to learn more, and to receive updates and news.
PRESS CONTACT:
Deborah Apsel Lang | (617) 495-7993 | kempnercommunications@harvard.edu